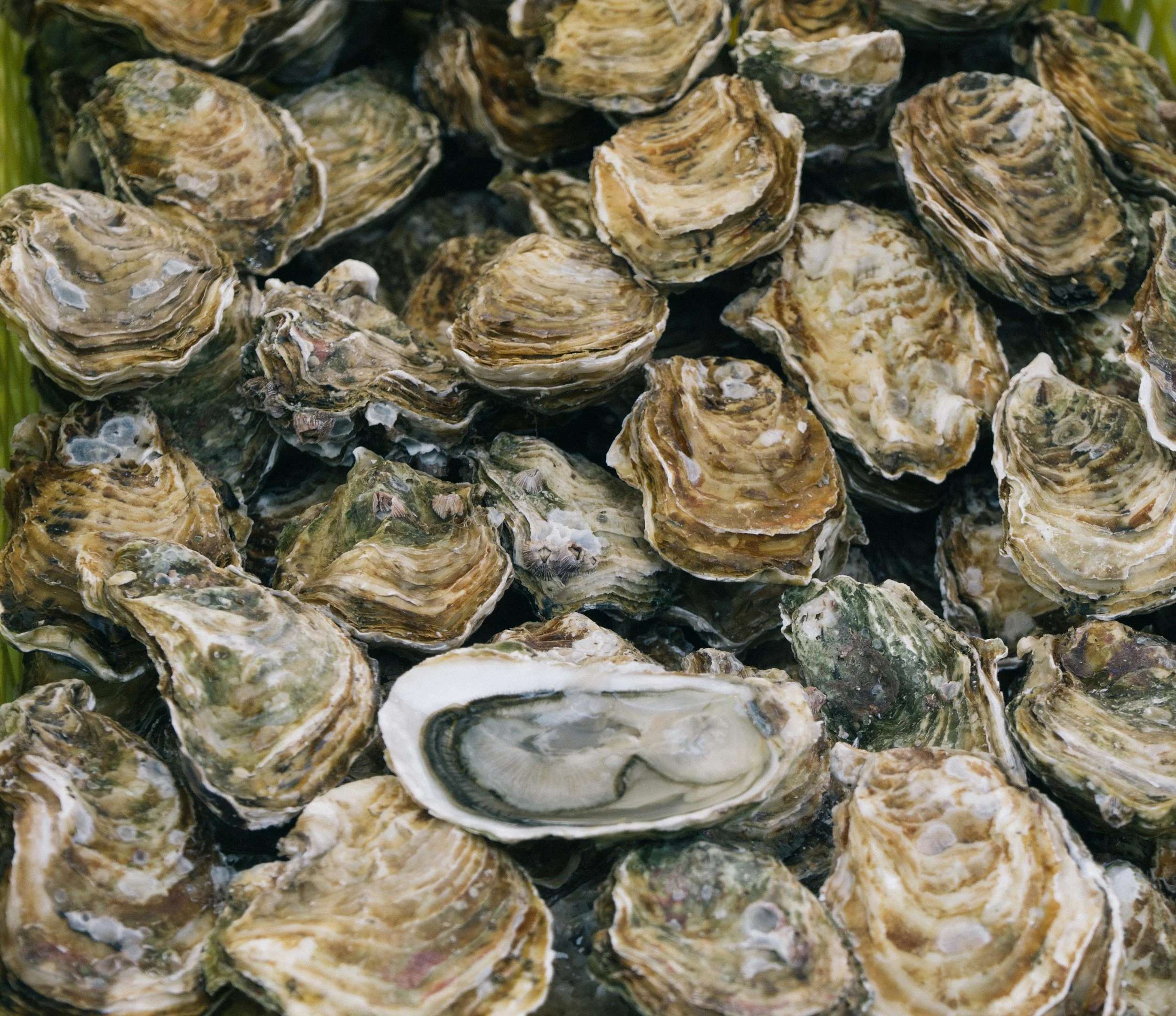
Make it stand out
Scientific Literature Review:
Eastern Oyster and Ribbed Mussel as Living Shoreline
REVIEW OF EASTERN OYSTER AND RIBBED MUSSEL SPECIES AS FUTURE LIVING SHORELINE INSTRUMENTS TO PREVENT COASTAL EROSION OF EASTERN U.S.A.
Alexander, Catherine
School of Marine and Atmospheric Sciences
Stony Brook University
ABSTRACT
This scientific literature review looks at temperature tolerance, filterable particle sizes, and retention efficiency of filtration in two Northeastern U.S. bivalve species. Crassostrea virginica, the eastern oyster, and Geukensia demissa, the ribbed mussel are judged as more resistant to changing environmental conditions caused by the greenhouse effect through three metrics of temperature tolerance, filterable particle size, and retention efficiency. This review identifies scientific literature to choose which species, Crassostrea virginica or Geukensia demissa, is a more appropriate future choice in living shoreline coastal erosion prevention projects. The scientific literature reviewed indicates that Crassostrea virginica have slightly higher filtration retention efficiency rates.
INTRODUCTION
Climate change is causing an increase in ocean temperature, ocean acidification, and more severe storms and precipitation. Oceans absorb heat from Earth’s warming climate, leading to an increase in saltwater temperatures. Earth’s warming atmosphere is creating more intense storms and greater precipitation, resulting in more polluted coastal waters from inland runoff and flooding. These climate change effects of increased water temperature and pollution are altering underwater climates. Temperature and filtration ability must be considered when reviewing and identifying which of the two selected bivalve species, either Crassostrea virginica (eastern oysters) or Geukensia demissa (ribbed mussels), will be most suitable for future living shoreline projects along the eastern U.S. coast. Both eastern oyster beds and ribbed mussel colonies are standard biological instruments for living shoreline projects and will be crucial for combating future coastal erosion and protecting productive estuary waters from sea level rise and increased storm surge. This study will identify either the Crassostrea virginica (eastern oyster) or the ribbed mussel (Geukensia demissa) as the more resilient species choice for future eastern U.S. intertidal living shoreline projects designed to combat coastal erosion from the climate change effects of sea level rise and increased storm surge.
Crassostrea virginica, the eastern oyster, is native to a range of habitats from Canada’s Gulf of St. Lawrence down to the Mexican Gulf (Eastern Oyster, 2018, p. 3). This filter feeder and keystone species lives in most estuaries attached to the Gulf and Atlantic in the United States and offers a host of ecosystem services to habitat including water filtration / concentration of pseudofeces, nutrient sequestration, and stabilization of surrounding habitats and shoreline (Eastern Oyster, 2018, p. 3). Geukensia demissa, the ribbed mussel, lives in mid and low intertidal estuaries, mostly in the southeastern USA, and is also native to coastal marine habitat from Canada’s Gulf of St. Lawrence to Texas (Coen & Walters, p. 2). Both bivalves filter particles through their gills and select particles to pass through their digestive systems or send particles back out without being digested as pseudofeces (Coen & Walters, p. 1). Both are native to fringing salt marsh and intertidal oyster reefs (Coen & Walters, p. 2). Ribbed mussels are known for attaching themselves to Spartina marsh grasses and oyster reef (Coen & Walters, p. 2). Unlike oysters, ribbed mussels that are dislodged can reattach, which gives them a species advantage, as they can more easily respond to disturbance (Coen & Walters, p. 2).
METHODS
I’m going to go about finding the answer to this question by doing a scientific literature review of the physiological resiliencies of the oyster and ribbed mussel species to evolving marine conditions from climate warming along the U.S. east coast. This scientific literature review is based on three metrics of temperature tolerance and filtration abilities. The metric of temperature will be the temperature tolerance range of each bivalve species. The metric of filtration will include size of particles filtered and filtration efficiency.
The temperature metric will be a review of the range of temperatures where each species can live and reproduce. Temperature range will be used to gage tolerance to future irregular fluctuating water temperatures caused by the greenhouse effect. Temperature will determine what latitudinal geographic areas each species could possibly survive in along the eastern U.S. coast from Maine to Texas.
The filtration metric will be a review of differing abilities of each species to filter the water column. The need for each species to successfully and efficiently filter seawater comes from climate change effects causing increasingly severe storms with intensified storm surge and increased precipitation causing runoff and flooding, all leading to water quality issues and ocean pollution. Inland runoff and flooding both result in water quality issues from various pollutants like sediment, debris, and agricultural fertilizers that cause low oxygen zones in estuaries and the ocean. The various filtration measurements for this metric will be the size of particles filterable by each species and efficiency of filtration.
FINDINGS
The first study review will be for the temperature tolerance metric of Crassostrea virginica. This 2016 study is of Lannig et al. collecting wild eastern oysters from Stump Sound, NC in the Fall of 2004 through the Winter of 2005 in seawater somewhere between 10 and 20 °C (2006, p. 279). The oysters were kept in artificial seawater in recirculating aerated tanks at 18°2 °C and 30 ppt salinity for 5 days to acclimate to new water temperatures (Lannig et al., 2006, p. 279). After 5 days the tank temperatures changed to 20, 24 and 28 °C (2006, p. 279). No oyster mortality occurred during the 10 days after temperature treatments were implemented (Lannig et al., 2006, p. 279). The temperature treatments increased by 2 °C day−1 until they reached their target temperatures (Lannig et al., 2006, p. 279). Later, 50g l−1 of Cadmium was added to, “half of the replicate tanks at each temperature” (Lannig et al., 2006, p. 279). The oysters were monitored for 40 days, except for the oysters in the 28 °C treatment exposed Cadmium (Lannig et al., 2006, p. 279). The oysters exposed to 28 °C and Cadmium had a 46% mortality rate, so monitoring of those subjects was discontinued after 20 days (Lannig et al., 2006, p. 279 & 281).
Lannig et al. studied the effects of temperature on Crassostrea virginica in combination with water pollutants to see if cadmium (Cd) pollution affects acclimation of Crassostrea virginica to changing temperature (2006, p. 278). Lannig et al. found zero mortality for control oysters after 30 days at 20 ◦C, “and only 4% and 25% mortality at 24 and 28 ◦C, respectively” (Lannig et al., 2006, p. 281). The oysters exposed to Cd at 20 and 24 ◦C had a 4% and 8% mortality rate,” respectively (Lannig et al., 2006, p. 281).
In a study conducted by Hans Riisgård and published in 1988, filtration efficiency and gill ciliary structure were studied in both Geukensia demissa (Atlantic ribbed mussels) and Crassostrea virginica (Eastern Oysters, p. 217). Geukensia demissa and Crassostrea virginica were both collected from intertidal zones within Georgia, USA in 1987 (Riisgård, 1988, p. 217). Riisgård chose to use the clearance method for measuring filtration efficiency in ribbed mussels and eastern oysters (1988, p. 220). The filtration rates in Riisgård’s 1988 study were measured at 27 to 29 degrees Celsius (p. 220). Riisgård used bacteria, 2 to 3 μm particles, 3 to 4 μm (Isochrysis galbana), and 5 to 9 μm particles (Cryptomonas sp.) of cultivated unicellular algae for measurement of retention efficiency (1988, p. 218). All algae used in the study were, “nearly spherical shape to assure reliable measurements of their diameter with the electronic particle counter” (Riisgård, 1988, p. 218). Particle concentration was measured with, “a Coulter Counter, Model TAII, with a population accessory plotter and a 100 μm aperture tube” (Riisgård, 1988, p. 218). Any bivalves that had not fully opened were not used in the study (Riisgård, 1988, p. 218).
In the literature, there has been some contradiction about whether oysters can keep relatively constant filtration rates while adapting to different temperatures, both high and low. In Riisgård’s 1988 study, both Geukensia demissa and Crassostrea virginica were able to adapt to both high and low temperatures while maintaining near constant filtration and metabolic rates (Riisgård, 1988, p. 220). A study done by Onorevole et al. in 2018, which will be fully reviewed later in this literature review, contradicted Riisgård, showing filtration rates of oysters that were proportional to temperature (2018, p. 243). In another study that will be fully reviewed later, Haven and Morales-Alamo published experiments in which Crassostrea virginica did not display significant changes in pumping rate varying temperatures ranging from 16 to 28 °C (Haven & Morales-Alamo, 1970, p. 253).
The majority of findings in these studies support the theory that bivalve, and specifically oyster, filtration rates are not significantly altered by changes in temperature. One disadvantage to the clearance method in Riisgård’s 1988 study was decreasing particle concentration during the time of the experiment, although the disadvantage can be minimized by adding new alga to maintain the particle concentration within a specific range (Riisgård, 2001, pp. 279-280). This was described in another article by Riisgård that was in 2001, 13 years after his 1988 bivalve study, on how differing methods of measuring filtration in bivalves can cause issues with conflicting data.
The scientific literature review of temperature tolerance of Geukensia demissa will be of a 1989 study conducted by Wilbur and Hilbish. In Wilbur and Hilbish’s 1989 study, Geukensia demissa physiological changes were measured to ribbed mussels in increased temperature conditions (p. 163). Wilbur and Hilbish collected Geukensia demissa from a South Carolina intertidal oyster reef and exposed the mussels to increased temperatures in a laboratory recirculating system that was simulating tidal cycles, microalgal feeding, and 30% salinity (pp. 161 & 163). Both air and water temperatures in the experiment were kept at 25 °C (Wilbur & Hilbish, 1989, p. 163). The Wilbur and Hilbish study showed ribbed mussel acclimation of physiological rates to a temperature increase from 15 °C to 25 °C, over a 28 day period (1989, p. 161).
G. demissa was able to acclimate, albeit differently depending on the physiological process, to the 10 °C increase in temperature (Wilbur & Hilbish, 1989, p. 168). Ribbed mussels in both the fed and starved groups of the experiment, “completely acclimated to aerial respiration rates within 28 days of a 10 °C elevation in temperature,” and were able to partially acclimate their aquatic respiration rates as well (Wilbur & Hilbish, 1989, p. 168). This study saw ribbed mussels reducing metabolic expenditures in response to a prolonged increase in temperature as well as adapting their other physiological rates to increase the animals’ energy (Wilbur & Hilbish, 1989, p. 169). Wilbur and Hilbish could not discern any direct effects of adjusted temperature on Geukensia demissa absorption efficiency (Wilbur & Hilbish, 1989, p. 169). Clearance rates increased with temperature and continued to increase as the mussels continued to be exposed to elevated temperature (Wilbur & Hilbish, 1989, p. 169). “Natural variation in environmental temperature is very high within populations of G. demissa. Ambient temperature may vary >15 °C within a single day… Even while submerged, average water temperatures varied by as much as 5 °C on successive tides… This study indicates that G. demissa is immune to short-term changes in temperature and improves its energy budget following prolonged increases in temperature” (Wilbur & Hilbish, 1989, p. 169).
Another study conducted by Jost and Helmuth, published in 2007, showed results that suggested that ribbed mussel mortality experiences a large increase during peak temperatures of 45 °C (Jost & Helmuth, 2007, p. 141). This analysis was conducted twice to make sure that body size was not an important variable.
A third study done by Virgin et al. in 2019 looked at the effects of temperature on larval biology of ribbed mussels (p. 1). Virgin et al.’s study supports the hypothesis that ocean temperatures 15 °C, “may be a major limiting factor for successful development of larvae in the field and so for the dynamics of northern populations of ribbed mussels” (2019, p. 1). Larval survival at temperatures of 19, 23, and 28 °C were high (Virgin et al., 2019, p. 1). At 15 °C, larval mortality was 2 times greater than mortality at 19 °C (Virgin et al., 2019, p. 1).
The literature review particle size metric for Crassostrea virginica includes a study conducted by Haven and Morales-Alamo that was published in 1970. Between 1964 and 1967, Haven and Morales-Alamo monitored filtration flow of natural particles in 6 groups of 12 oysters each (p. 254). The individual experiments showed consistent results and were all designed with Crassostrea virginica being held in troughs of flowing water (Haven & Morales-Alamo, 1970, pp. 248 & 253). The particle sizes studied were between the sizes of 1.0 and 12.0 μm (Haven & Morales-Alamo, 1970, p. 250). The troughs simulated natural environmental conditions and used constantly flowing water to avoiding particle recycling by the oysters (Haven & Morales-Alamo, 1970, pp. 248 & 250). The seawater used in each experiment was pumped from the York River, 90 meters offshore and the oysters were sourced from the James River in Virginia, where there are no known oyster diseases (Haven & Morales-Alamo, 1970, p. 250). The water particles were measured with a Model B Coulter Electronic Particle Counter (Haven & Morales-Alamo, 1970, p. 251). The oysters were 2 years of age, weighed a wet meat average weight of 4.8 g, and an average of 50 mm in height (Haven & Morales-Alamo, 1970, pp. 250-251).
During their monitoring of Crassostrea virginica, the filtration flow of the water holding the oysters was 1.01 l/min (Haven & Morales-Alamo, 1970, p. 254). In Haven and Morales-Alamo’s study, Crassostrea virginica increased the filtration of particles as particle size increased from 1.0 to between 2.0 and 4.0 μm (p. 253). The oysters fed mostly on particles between 1.0 to 2.0 μm in size from water with, “an average of over 1 billion,” particles per liter (Haven & Morales-Alamo, 1970, p. 254). The number of particles decreased quickly with size (Haven & Morales-Alamo, 1970, p. 254). This study did not measure total solids consistently. On average, 18% of Particles filtered by the oysters were between 1 and 2 μm. Particle removal increased in Crassostrea virginica to 53% for particles between 3.0 and 4.0 μm (Haven & Morales-Alamo, 1970, p. 254). For particles larger than 4.0 μm, Haven and Morales-Alamo found no evidence of variation in removal efficiency dependent on particle size (Haven & Morales-Alamo, 1970, p. 254).
Haven and Morales-Alamo used 6 studies of groups of 12 Crassostrea virginica subjects for their experiment, totaling 72 Crassostrea virginica monitored subjects. The water used in the study was pumped in from the York River, which may have helped the experiment simulate more realistic environmental conditions for the oysters as far as particle types and sizes in seawater. This study is half a century old and cites many other scientific works published between 1928 and 1967 to bolster their own study. We can infer that the water quality that the oysters were subject to in this study may not reflect water quality in the York River today. We cannot infer that Haven and Morales-Alamo’s results represent average species behavior for all Crassostrea virginica, although their consistent results among each of the 6 groups could be used to infer that Crassostrea virginica will behave in a similar way if this same experiment is repeated with similarly-sized, similarly-aged, healthy oysters by simulating the same conditions as in the original study.
The 1988 study by Riisgård that was earlier reviewed for Crassostrea virginica temperature tolerance also provides data the sizes of particles filtered by Crassostrea virginica. To review, Hans Ulrik Riisgård studied filtration efficiency and gill ciliary structure in both Geukensia demissa (Atlantic ribbed mussels) and Crassostrea virginica (eastern oysters) (Riisgård, 1988, p. 217). Riisgård used bacteria, 2 to 3 μm particles, 3 to 4 μm (Isochrysis galbana), and 5 to 9 μm particles (Cryptomonas sp.) of cultivated unicellular algae for measurement of retention efficiency (1988, p. 218). All algae used in the study were, “nearly spherical shape to assure reliable measurements of their diameter with the electronic particle counter” (Riisgård, 1988, p. 218). “The spectrum of particle retention was found by expressing the clearance of the different sized particles relative to the mean of the 2 highest clearance rates measured” (Riisgård, 1988, p. 218).
Scientific literature for the particle size metric for Geukensia demissa were limited. Riisgård’s 1988 study, reviewed earlier for other metrics like oyster temperature and oyster particle size in this review, reports that, “particles above 4 μm are completely retained in bivalves that possess large laterofrontal cirri (i.e. Geukensia demissa…) whereas only particles larger than 5 to 6 μm are entirely retained in Crassostrea virginica, which has small laterofrontal cirri” (p. 220). Murphy et al.’s study published in 2019 cites Riisgård’s 1988 study, also reported Geukensia demissa as having gills with large latero-frontal cirri, allowing ribbed mussels to more efficiently filter smaller particles that are larger than 4 μm when compared to Crassostrea virginica (pp. 353-354). Murphy et al.’s study also cites Riisgård’s 1988 study showing that Crassostrea virginica can only entirely retain particles larger than 5 to 6 μm (2019, pp. 353-354).
Riisgård refers to a 1982 study of gill function in Geukensia demissa to show inconsistencies between his 1988 results and the 1982 study. The 1982 study that Riisgard cites says that, “according to the ‘filter theory’, the size threshold for particles 100% retained should be… considerably lower than 4 μm,” for Geukensia demissa (Riisgård, 1988, p. 220). Riisgård’s results showed efficient retainment of 4 μm particles and 70% retainment efficiency of 2 μm particles (Riisgård, 1988, p. 220).
A study of Geukensia demissa short-term grazing experiments, published in 2009 by Lonsdale et al., were conducted from 2003 to 2004 to find total grazing pressure from bivalves and microzooplanton on phytoplankton (p. 263). The purpose of this study was to measure natural planktonic assemblages in two locations in the Peconic Bay estuary, specifically West Neck Bay on Long Island, to understand how the bivalve community and zooplankton community affect phytoplankton mortality. Lonsdale et al. studied grazing of 3 different bivalve species living in the Peconic Bay estuary: Geukensia demissa, Mya arenaria (soft-shell clam), and Mercenaria mercenaria (hard clam) (2009, p. 263).
This study was designed by choosing two very similar embayment field sites: a had clam spawning sanctuary and a control site, in West Neck Bay, Shelter Island (Lonsdale et al., 2009, p. 264). In 2003 both sites were monitored, but only the sanctuary site was monitored in 2004 and had a much larger abundance of Mya arenaria during the second year (Lonsdale et al., 2009, p. 264). Both sites had an abundance of Geukensia demissa along the edges (Lonsdale et al., 2009, p. 264). Lonsdale et al. monitored temperature, salinity, bay volume, tidal flushing, residence time, water depth, perimeter and area at each site (2009, p. 265). They did bi-weekly to experiments on bivalve feeding from May to October during each of the two years, collecting data on the total phytoplankton community and measuring feeding of picoplankton and microplankton in plastic buckets at each study site (Lonsdale et al., 2009, pp. 265-266). The buckets held different bivalves with only one bivalve species per bucket and held 3 to 4 Geukensia demissa in each bucket (Lonsdale et al., 2009, p. 266). Each experimental treatment / control tested 3 different buckets and plankton abundance was studied in each bucket using a FACScalibur (BD) flow cytometer (Lonsdale et al., 2009, p. 266). Water samples taken were, “processed using standard settling techniques and inverted light microscopy” cytometer (Lonsdale et al., 2009, p. 266).
Results for this study recognized Geukensia demissa as, “more efficient at clearing particles in the 0.5 to 2.5 μm range than several other bivalve species… and equally capable of ingesting carbon from small bacteria (<1 μm estimated spherical diameter ESD) and heterotrophic flagellates (3 to 5 μm ESD)… Clearance rates… on phytoplankton (i.e. Isochrysis galbana, 4.8 μm ESD) and benthic diatoms (15 μm ESD), however, were higher for ribbed mussels compared to bacteria and heterotrophic protists” (Lonsdale et al., 2009, p. 277). Lonsdale et al.’s field research showed that, “in a natural plankton assemblage… ribbed mussels removed Synechococcus (0.5 to 1 μm ESD) and photosynthetic picoeukaryotes (1 to 3 μm)” (Lonsdale et al., 2009, p. 277). These results can be used for the ribbed mussel particle size metric for this scientific literature review, but doesn’t provide data on the full range of particle sizes filterable by Geukensia demissa. This review will refer back to Lonsdale et al.’s 2009 study for Geukensia demissa’s nutrient filtration metric. There is a large research gap in particle size (μm) range that can be filtered by ribbed mussels, as well as species preference to particle size.
For Crassostrea virginica’s filtration retention efficiency metric, the 1988 study by Riisgård will be reused; It has been reviewed for both the Crassostrea virginica temperature and particle size metrics is also relevant to the eastern oyster’s filtration retention efficiency metric, as it provided some data for all three of this scientific literature review’s metrics. Riisgård states that, “the capacity of the bivalve pump can be exploited only in undisturbed bivalves, and that the pump potential presumably constitutes an evolutionary adaptation to the prevailing food concentrations in the environment… Therefore, the filtration rate capacities measured in the present work may be regarded as estimates of normal pumping rates in undisturbed natural bivalves” (1988, p. 222). This study showed that both Geukensia demissa and Crassostrea virginica particle retention rates were directly related to dry weight of the soft parts of the bivalve and that retention rate increased with an increase in dry weight of the animal (Riisgård, 1988, p. 219).
Riisgård’s results showed both Geukensia demissa and Crassostrea virginica completely retaining particles above 4 μm. Crassostrea virginica can entirely retain particles larger than 5 to 6 μm (Riisgård, 1988, p. 217). The retention efficiency for smaller particles decreases for Crassostrea virginica (Riisgård, 1988, p. 217). “Below 5 μm the retention efficiency gradually decreased to 50% for 2 μm particles in C. virginica” (Riisgård, 1988, p. 217). Figure 1 (below) was borrowed from this study and shows filtration retention efficiency rates for both Geukensia demissa and Crassostrea virginica (Riisgård, 1988, p. 218). In this study, Crassostrea virginica had the highest filtration retention rates when compared to Geukensia demissa (Riisgård, 1988, p. 219).
Fig.1- Particle retention efficiency as a function of particle diameter in Geukensia demissa and Crassostrea virginica. Each value is the mean and range of one measurement on each of 2 to 3 individuals as indicated by the figures in parentheses (Riisgård, 1988, p. 218).
Riisgård used the clearance method to measure filtration retention efficiency in eastern oysters. A disadvantage to the clearance method is decreasing particle concentration during the time of the experiment, although the disadvantage can be minimized by adding new alga to maintain the particle concentration within a specific range (Riisgård, 2001, pp. 279-280). “Retention efficiency in… Crassostrea virginica may be adjusted in response to low or high algal concentrations” (Riisgård, 1988, p. 220). In an article written by Riisgård in 2001, he explains that filtration rate differences between various authors is not necessarily an indication of unreliable data, but instead may be due to oyster and other bivalve health and/or from environmental conditions (Riisgård, 2001, p. 288). Previous studies referenced in Riisgård’s study indicate that, “the bivalves studied probably do not change their retention efficiency in response to particle concentrations” (Riisgård, 1988, p. 220).
There was an obvious gap in research done on filtration retention efficiency, as this study was the only one that I could find. Much more needs to be studied on this subject, preferably in natural marine habitats, to further show how filtration retention efficiency changes for Crassostrea Virginica when exposed to certain environmental stressors.
Unfortunately, scientific literature on the filtration retention efficiency metric for Geukensia demissa is also lacking. Riisgård’s 1988 study was the only peer-reviewed article that I could find on the topic. The results of that study showed that eastern oysters had a higher retention rate, but that the retention rates of ribbed mussels were close to the retention rates measured for Crassostrea virginica (p. 219). It should be noted that as gill area increased for Geukensia demissa, “at the same rate as filtration” (Riisgård, 1988, p. 217). In 1988 the study, G. demissa’s filtration efficiency decreased for particles smaller than 4 μm (Riisgård, p. 217). “Below 4 μm the retention efficiency decreased gradually”, to 70 % efficiency for 2 μm particles (Riisgård, 1988, pp. 219-220). The filtration rate of Geukensia demissa seems to be, “directly correlated to gill area” (Riisgård, 1988, p. 219).
There is also a huge gap in research for retention efficiency of Geukensia demissa. Much more also needs to be studied on this subject for Geukensia demissa, preferably in natural marine habitats, to further show how filtration retention efficiency changes when exposed to certain environmental stressors.
IMPLICATIONS OF FINDINGS
For this topic of identifying resiliency in northeastern American bivalves to warming ocean temperatures and increased water pollution from increasingly regular and intensifying storms, there are so many research gaps that it’s difficult to point out the specifics. Every metric that I used had huge research gaps. It was unclear which species was most temperature tolerant, as not enough studies have been done on the limits of low and high temperature conditions causing mortality in both Crassostrea virginica and Geukensia demissa. Originally, I had planned to include pH tolerance as a metric for this scientific review to help identify which species would be more tolerant to ocean acidification, but there wasn’t enough literature to even include it as a metric due to the fact that I couldn’t find any scientific peer-reviewed articles testing pH tolerance in Geukensia demissa. Particle sizes filterable was a metric that was difficult to find studies to review for. Temperature, strangely, was also lacking in research that just focused on temperature tolerance without other research questions and experiments being intertwined in each study. Filtration retention efficiency was the only metric out of all of the three chosen metrics in this review that had enough easily accessible peer-reviewed articles for each species to do a proper species comparison. Crassostrea virginica and Geukensia demissa responded similarly to temperature and live within the same native geographic range, showing that they are comfortable with similar water temperature conditions.
This scientific literature review included only peer-reviewed scientific articles, which resulted in a lack of overall data / research to review. Non-peer-reviewed articles and websites online provide data for each of the chosen metrics, but this is scientific literature review on peer-reviewed studies and attempts to show gaps in scientific literature.
CONCLUSION
This review provides minimal insight into which species is more viable and resilient for mass production and planting/building as living shoreline along the U.S. eastern coast to combat coastal erosion caused by the greenhouse effect, as there are so many research gaps in this new field of simulating expected climate change conditions for marine species to gain insight into strengths and weaknesses of different similar species to increasing water temperature and increased water pollution from increasing storm surge as well as increasing and intensifying storms.
This review does not account for balancing of marine trophic dynamics or for ensuring food availability for the bivalve species released in sessile living shoreline marine installations. Trophic dynamics and food availability will have to be assessed for each potential bivalve planting/building location through an environmental assessment impact report before being site-approved and implemented, or denied, as a new living shoreline installation.
REFERENCES:
Chu, F.-L. E., & La Peyre, J. F. (1993). Perkinsus marinus susceptibility and defense-relate activities in eastern oysters Crassostrea virginica: temperature effects. Diseases of Aquatic Organisms, 16, 223-223.
Coen, L., & Walters, K. (2005). Ribbed Mussel. South Carolina State Documents Depository.
Ellington, W. R. (1983). The extent of intracellular acidification during anoxia in the catch muscles of two bivalve molluscs. Journal of Experimental Zoology, 227(2), 313-317. doi:10.1002/jez.1402270215
Galimany, E., Rose, J. M., Dixon, M. S., & Wikfors, G. H. (2013). Quantifying Feeding Behavior of Ribbed Mussels (Geukensia demissa) in Two Urban Sites (Long Island Sound, USA) with Different Seston Characteristics. Estuaries and Coasts, 36(6), 1265-1273. doi:10.1007/s12237-013-9633-0
Haven, D. S., & Morales-Alamo, R. (1970). FILTRATION OF PARTICLES FROM SUSPENSION BY THE AMERICAN OYSTER CRASSOSTREA VIRGINICA. The Biological Bulletin, 139(2), 248-264. doi:10.2307/1540081
Jordan, T. E., & Valiela, I. (1982). A nitrogen budget of the ribbed mussel, Geukensia demissa, and its significance in nitrogen flow in a New England salt marsh1. Limnology and Oceanography, 27(1), 75-90. doi:10.4319/lo.1982.27.1.0075
Jost, J., & Helmuth, B. (2007). Morphological and ecological determinants of body temperature of Geukensia demissa, the Atlantic ribbed mussel, and their effects on mussel mortality. The Biological Bulletin, 213, 141+. Retrieved from https://link.gale.com/apps/doc/A170864108/AONE?u=sunysb&sid=AONE&xid=819fcc57
Lannig, G., Flores, J. F., & Sokolova, I. M. (2006). Temperature-dependent stress response in oysters, Crassostrea virginica: Pollution reduces temperature tolerance in oysters. Aquatic Toxicology, 79(3), 278-287. doi:10.1016/j.aquatox.2006.06.017
Lonsdale, D., Cerrato, R., Holland, R., Mass, A., Holt, L., Schaffner, R., . . . Caron, D. (2009). Influence of suspension-feeding bivalves on the pelagic food webs of shallow, coastal embayments. Aquatic Biology, 6, 263-279. doi:10.3354/ab00130
Mitchell, M., & Bilkovic, D. M. (2019). Embracing dynamic design for climate‐resilient living shorelines. Journal of Applied Ecology, 56(5), 1099-1105. doi:10.1111/1365-2664.13371
Murphy, A. E., Kolkmeyer, R., Song, B., Anderson, I. C., & Bowen, J. (2019). Bioreactivity and Microbiome of Biodeposits from Filter-Feeding Bivalves. Microbial Ecology, 77(2), 343-357. doi:10.1007/s00248-018-01312-4
Onorevole, K. M., Thompson, S. P., & Piehler, M. F. (2018). Living shorelines enhance nitrogen removal capacity over time. Ecological Engineering, 120, 238-248. doi:https://doi.org/10.1016/j.ecoleng.2018.05.017
Pales Espinosa, E., Allam, B., & Ford, S. E. (2008). Particle selection in the ribbed mussel Geukensia demissa and the Eastern oyster Crassostrea virginica: Effect of microalgae growth stage. Estuarine, Coastal and Shelf Science, 79(1), 1-6. doi:https://doi.org/10.1016/j.ecss.2008.02.022
Riisgård, H. (2001). On measurement of filtration rate in bivalves-the stony road to reliable data: review and interpretation. Marine Ecology Progress Series, 211, 275-291. doi:10.3354/meps211275
Riisgård, H. U. (1988). Efficiency of particle retention and filtration rate in 6 species of Northeast American bivalves. Marine ecology progress series. Oldendorf, 45(3), 217- 223.
Smith, C. S., Rudd, M. E., Gittman, R. K., Melvin, E. C., Patterson, V. S., Renzi, J. J., . . . Silliman, B. R. (2020). Coming to Terms With Living Shorelines: A Scoping Review of Novel Restoration Strategies for Shoreline Protection. Frontiers in Marine Science, 7. doi:10.3389/fmars.2020.00434
Virgin, S., Sorochan, K., Metaxas, A., & Barbeau, M. (2019). Effect of temperature on the larval biology of ribbed mussels (Geukensia demissa) and insights on their northern range limit. Journal of Experimental Marine Biology and Ecology, 512. doi:10.1016/j.jembe.2018.11.006
Wilbur, A. E., & Hilbish, T. J. (1989). Physiological energetics of the ribbed mussel Geukensia demissa (Dillwyn) in response to increased temperature. Journal of Experimental Marine Biology and Ecology, 131(2), 161-170. doi:https://doi.org/10.1016/0022-0981(89)90005- 1